More news
- Focus on the global coatings market: Global coatings market outlook
- Innovative coatings mitigate effects of deepening climate change
- View from the UK: Navigating chemical policy and sustainability
- Focus on adhesives: Unveiling unbreakable bonds – Testing redefines physical strengt...
- Focus on adhesives: Henkel and Covestro collaborate for sustainability of engineered wood ...
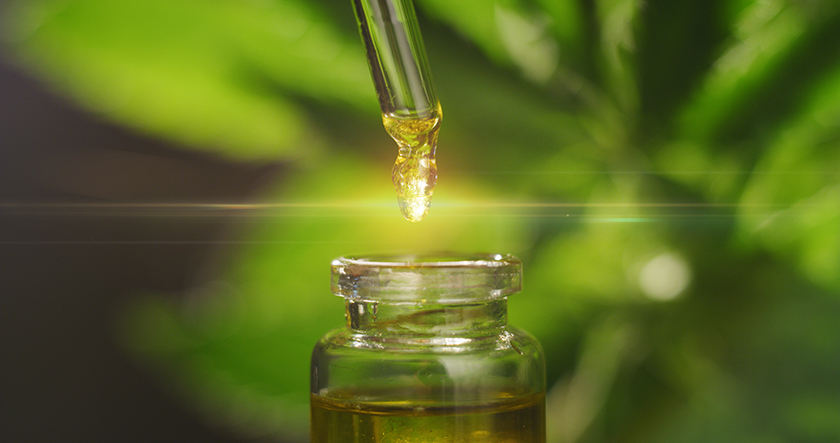
In recent years, many efforts are being devoted to the development of new materials that originate from renewable resources. Natasa Cuk, Miha Steinbücher, Nejc Vidmar, Martin Ocepek and Peter Venturini, Helios Resins, discuss how polyesters are one of the most important classes of such materials and several bio-based monomers are available for their synthesis. In this work, the development of fully bio-based and solvent-free polyester polyol used for two-component polyurethane coatings on industrial scale is presented
Fossil-based raw materials were substituted with bio-based alternatives that are commercially available on a large scale. Properties of polyols and coatings were determined and measured. Polyols were characterised by the determination of acid number, hydroxyl number, glass transition temperature and refractive index, and measurement of viscosity, colour and molecular weight. Coatings were characterised by the determination of mechanical properties, such as hardness, elasticity and impact resistance, and the measurement of optical properties such as gloss, haze, distinctness of image (DOI) and reflected image quality (RIQ) and weathering resistance.
Three variations of bio-based polyol were synthesised, then the most suitable version was validated in a clear coat. The results showed that the properties of the bio-based polyol and coating met the requirements and were comparable to the properties of the synthetic counterpart. Results indicate that this newly developed, 100% bio-based and solvent-free polyol can be used as a drop-in replacement for synthetic polyol. Furthermore, this work implies that the supply chain is established, which allows the green transition in the paint industry.
Nowadays, much effort in the paint and coating industry is being invested in the development of bio-based materials, as the conversion of biomass and bio-based ingredients into useful products for paints and coatings offers considerable and sustainable growth potential.
In recent years, the potential replacement of fossil fuels to produce appropriate monomers using inexpensive and renewable starting materials, such as cellulose, starch, lignin, proteins, vegetable oils and fats, is increasingly being explored. These bio-based materials can be used to make bio-based polyester polyols, polyurethane dispersions, modified acrylic dispersions, polyacrylate polyols, polyurethanes, epoxy coatings, powder coatings, UV coatings and printing inks, architectural coatings, wood and plastic coatings, paper coatings, films and others.
Bio-based polyesters are one of the most important classes of polymers for coatings. There are many monomers available for their synthesis, including acids (succinic acid, sebacic acid, adipic acid, levulinic acid, gluconic acid, terephthalic acid, 2,5-furandicarboxylic acid, itaconic acid, lactic acid, 3-hydroxypropionic acid, etc.), and glycols (ethylene glycol, 1,3-propanediol, 1,4-butanediol, 1,5-pentanediol, isosorbide, xylitol, sorbitol, glycerol, etc.).
There are two main approaches when introducing bio-based raw materials, (a) the conventional substance is substituted with a bio-based alternative, for example synthetic terephthalic acid can be replaced with 2,5-furandicarboxylic acid (FDCA) derived from biomass, and (b) the so-called drop-in approach, where a fossil-fuel derived raw material is substituted with the chemically identical substance which is derived from biomass, e.g., synthetic adipic acid can be replaced with bio-based adipic acid. In addition, a biomass balance approach can be used. Since 2021, our company is ISCC (International Sustainability and Carbon Certification) PLUS certified and the mass balance approach is used for raw materials, such as neopentyl glycol, trimethylol propane and pentaerythritol. The ISCC PLUS certification scheme uses the mass balance method to determine the content of bio-based or recycled material. This approach can facilitate a gradual increase of renewable and recycled raw materials by enabling the use of existing infrastructure and equipment instead of constructing separate plants and value chains for renewable and/or recycled raw materials. It also ensures product sustainability, as certification schemes have been developed to meet the requirements of the EU Renewable Energy Directive (RED).
READ MORE:
Focus on Sustainability: The power of collaboration – Uniting for sustainable success
The use of polyols in industrial organic coatings is regulated by Directive 2010/75/EU of the European Parliament and the Council of 24 November 2010 on industrial emissions, which limits the emissions of volatile organic compounds (VOCs) due to the use of organic solvents in certain activities and installations. One of the practically useful technologies for meeting the requirements of this directive are high-solid coatings. The directive does not prescribe a maximum content of VOCs in the coating, nevertheless, in large industrial paint shops the coating should have at least 72.7% of non-volatile matter. This also applies to polyurethane topcoats and single-layer coatings. Such coatings are usually prepared based on acrylic polyols, but formulators often decide to include polyester polyol as a binder in the formulation as well to increase solid content. For this purpose, low-viscosity solvent-free polyester polyols with a viscosity between 750 and 1000 mPa·s, a glass transition temperature between −70 and −50 °C and a hydroxyl number between 240 and 290 mg KOH/g are especially suitable.
The performance of bio-based materials needs to match the performance of fossil fuel-based materials, therefore the balance of bio-based content against performance and costs needs to be achieved. Polyester polyol that was 100% bio-based and solvent-free polyol was developed to be used as a replacement for synthetic polyester polyol in combination with an acrylic polyol in high-solid two-component polyurethane protective coatings for metal surfaces. The bio-based raw materials used in this work are already being produced on a large scale and were obtained from commercially available sources. Bio-based succinic acid and sebacic acid were used to replace adipic acid, bio-based 1,3-propanediol was used to replace 1,6-hexanediol and propylene glycol, and bio-based neopentyl glycol was used according to ISCC Plus mass balance approach to replace fossil-based neopentyl glycol. The biobased polyester polyol can thus be produced on an industrial scale using the equipment depicted in Figure 1. The properties of this newly developed bio-based polyester polyol and model coatings are comparable to the properties of its synthetic counterpart and comply with the requirements.
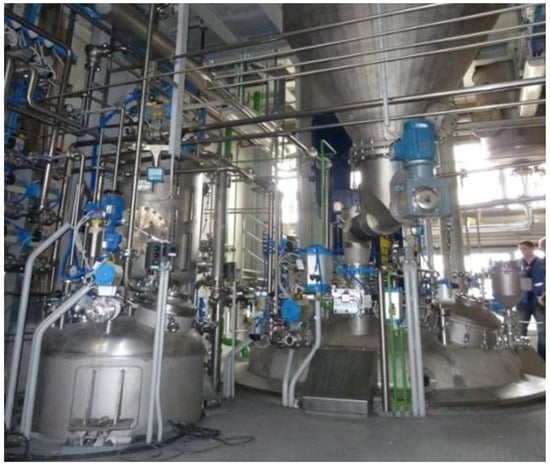
Materials and methods
Materials
Polyol synthesis
The synthesis of the polyol was carried out in a 6 L three-neck glass reactor, equipped with a mechanical stirrer, thermometer, condenser and external heating. The reactor was charged with raw materials and purged with nitrogen. The mixture was heated with constant stirring up to 210 °C. During the reaction, acid value and viscosity were checked and when the required values were reached, the reaction was finished. Afterward the resin was cooled down to room temperature and filtered through 190µm filter.
Four polyester polyols were synthesised. Synthetic polyol (PE-SYN), which is already commercially produced, was used as a reference and compared to the three bio-based polyols where synthetic raw materials were replaced with bio-based alternatives. Neopentyl glycol was substituted with bio-based neopentyl glycol using ISCC Plus mass balance approach, 1,6-hexanediol and propylene glycol were substituted with bio-based 1,3-propanediol and adipic acid was replaced with bio-based succinic acid and sebacic acid. The following three variations of adipic acid replacement were used in the formulation: (a) 100% replacement with bio-based succinic acid (PE-BIO-Succ), (b) 100% replacement with sebacic acid (PE-BIO-Seb) and (c) a replacement with a combination of bio-based succinic and sebacic acid at the molar ratio of 1:1 (PE-BIO-Succ+Seb).
Resin characterisation
Acid number determination
The acid number was determined according to the SIST EN ISO 2114 standard, as follows: a 0.4g of sample was weighed in a 250mL Erlenmeyer flask and dissolved in 50mL of solvent mixture. The solvent mixture consisted of xylene and ethanol (2:1 v/v). Then, 0.5 mL of phenolphthalein indicator solution (1% in ethanol) was added and titrated with 0.1 N KOH solution in methanol, to the equivalent point. The acid number (mg KOH/g of the sample) was calculated using Equation (1), as follows:
here, V is the volume of the potassium hydroxide solution required for the titration of the sample (mL), M is the molarity of the potassium hydroxide solution and m is the amount of the sample (g) being analysed.
Viscosity measurement
Hydoxyl number determination
The hydroxyl number of the resin was determined according to the SIST EN ISO 4629 standard as follows: 1.0g of sample was weighed in a 250mL Erlenmeyer flask and dissolved in 5mL of ethyl acetate. Then, 5mL of acetylating reagent was added. The mixture was heated in a water bath at 50°C for 45min and then cooled down to room temperature. After cooling the 10mL mixture of pyridine and water (3:1 v/v) was added and left at room temperature for 5min. Then, 60mL of a neutralized mixture of toluene and n-butanol (1:2 v/v) and 0.5mL of phenolphthalein indicator solution (1% in ethanol) was added and titrated with 0.5 N KOH solution in methanol to the equivalent point. The hydroxyl number (mg KOH/g of the sample) was calculated using Equation (2).
Here, A is the volume of the potassium hydroxide solution required for the titration of sample (mL), B is the volume of the potassium hydroxide solution for the titration of blank solution (mL), M is the molarity of the potassium hydroxide solution and w is the amount of the sample (g) being analysed.
How to use starting point formulations for easier formulating of acrylic waterborne paints
Colour measurement𝐴𝑐𝑖𝑑 𝑛𝑢𝑚𝑏𝑒𝑟=𝑉×𝑀×56.1𝑚
𝐻𝑦𝑑𝑟𝑜𝑥𝑦𝑙 𝑛𝑢𝑚𝑏𝑒𝑟=(𝐵−𝐴)×𝑀×56.1𝑚+𝑎𝑐𝑖𝑑 𝑛𝑢𝑚𝑏𝑒𝑟
READ MORE:
Gel permeation chromatography (GPC)
Glass transition temperature (Tg)
Refractive index
Validation of polyols in coatings
Synthetic polyol (PE-SYN) and its bio-based version (PE-BIO-Succ+Seb) were mixed with our acrylic polyol (1:5m/m) and crosslinked with solvent free low viscosity polyisocyanate based on HDI trimer. The amount of polyisocyanate needed was calculated based on the hydroxyl number of the resin mixture as determined and calculated in Section 2.3.3. and NCO content of the polyisocyanate provided by the producer.
The mixture of polyols and polyisocyanate was applied to the CRS panel of 0.8mm thickness (Q-Panel R-36, Q-Lab Deutschland GmbH, Saarbrücken, Germany) with a manual film applicator for determination of mechanical properties. For the determination of optical properties, the mixture thinned with a commercial polyurethane grade thinner was applied using SATA jet 5000 B RP spray gun and 1.2mm nozzle on precoated 10 × 15 cm CRS panels (Ernst Sauter AG, Meßstetten, Germany). All films were cured at 60°C for 48hr.
Thickness of the film
Elasticity of the film
Impact resistance
Optical properties
Weathering
A UV-B test, according to ISO 16474-3, Method C, cycle no. 4, was performed using a QUV UV tester (Q-Lab Deutschland GmbH). Film degradation was monitored by changes in the coatings’ IR spectra. The spectra were recorded using a Thermo Nicotet Nexus 6700 FT-IR spectrometer (Waltham, MA, USA). Photo oxidation values (POV) were calculated using methodology devised by Gerlock et al. as a ratio between combined -NH and -OH absorbances and -CH absorbance. -NH and -OH absorbances were integrated in the entire 4000–2000 cm−1 region with subtracted -CH absorbance. Initial -NH and -OH absorbances were subtracted for subsequent measurements for POV calculations.
Hardness of the film
READ MORE:
Results and discussion
Properties of the polyols
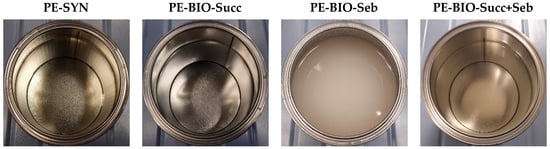
All synthesised polyols had an acid number, hydroxyl number and colour in the range as required, whereas viscosity was much higher for polyols where 100% bio-based succinic acid and 100% sebacic acid were used as a replacement for adipic acid. The requirement for the viscosity of the polyol is 750–1000mPa·s, while the viscosity of the polyol with 100% bio-based succinic acid reached the value of 2340 mPa·s and the viscosity of the polyol with 100% sebacic acid increased up to 2700 mPa·s. The combination of bio-based succinic acid and sebacic acid at a molar ratio of 1:1 as a replacement for adipic acid resulted in the viscosity of 960 mPa·s, which complies with the requirement and is comparable to synthetic polyol.
As can be seen from the results for the polyol with 100% bio-based succinic acid, the Tg of the crosslinked polyol increased, along with the viscosity, and was approximately 15 °C higher compared to other polyols, while molecular weight was comparable to standard polyol and is in agreement with the requirements. Compared to 1,6-hexanediol in PE-SYN and sebacic acid in PE-BIO-Seb succinic acid in PE-BIO-Succ has a shorter chain which has lower mobility and therefore gives more stiff products. Consequently, the viscosity and Tg of the polyol are higher when 100% succinic acid is used to replace adipic acid.
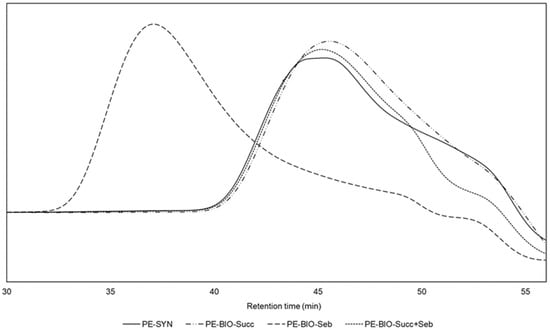
Properties of the coatings
As the synthesised polyester polyols are primarily meant as a modifier of acrylic polyols, we tested their basic mechanical and optical properties in model two-component polyurethane clear coats both as sole polyol binders (CC1-1 and CC1-2) and in a mixture with one of our commercially available acrylic polyols (CC2-1 and CC2-2). We decided to keep the formulations of all the model coatings simple and to avoid the use of additives. Such formulations may lead to lower performance in comparison to complexly formulated commercial 2-component polyurethane clear coats. At the same time, they allow for the potential differences between both polyester polyols to show more clearly. Use of levelling additives or HALS and UV absorbers may mask the difference in optical properties and weathering, respectively.
The difference between the pairs of materials (CC1-1, CC1-2 and CC2-1, CC2-2) usually lies within the repeatability limit of the test method used or is very close to it. As polyester polyol often acts as an elastifier when applied together with acrylic polyols, we find it significant that there was no difference in elasticity and impact resistance between CC2-1 and CC2-2.
Probably the most important property of a practical clear coat is weathering resistance. As both the PE-SYN and the acrylic polyol are well known for their excellent weathering resistance, it was decided to test the weathering resistance in CC1-1 and CC1-2 as the large amount of acrylic polyol in CC2-1 and CC2-2 may well hide the potential difference between the polyester polyols. Previous work by Ogorevc showed high the resilience of PE-SYN to UV-A and daylight filtered xenon irradiation. The UV-B test, according to ISO 16474-3, method C, cycle no. 4, on the other hand, produced measurable degradation. Subsequently, we used the same method of exposure in durations of 250, 500 and 1000 h. The POV derived from IR spectra, as seen in Figure 4, was used for the assessment of the results. They are presented in Figure 5.
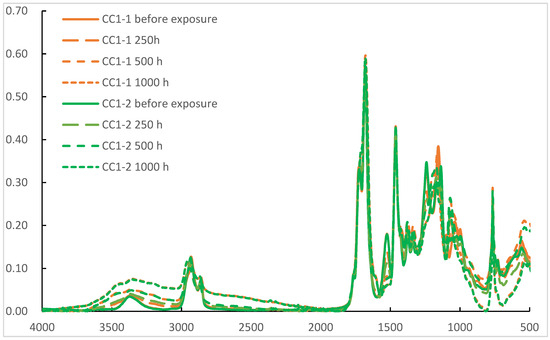
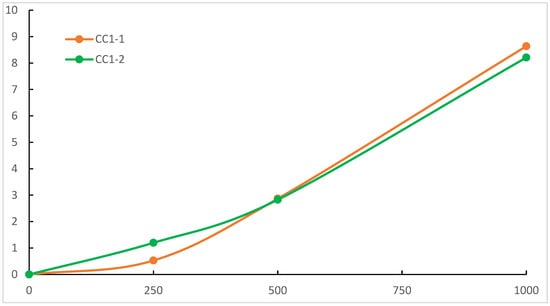
Conclusions
In this research, a fully bio-based version of synthetic polyester polyol was successfully synthesised to be used in combination with acrylic polyol for high-solid two-component polyurethane protective coatings for metal surfaces. Synthetic raw materials were substituted with their bio-based alternatives.
Neopentyl glycol was replaced with bio-based neopentyl glycol using ISCC Plus mass balance approach, 1,6-hexanediol and propylene glycol were substituted with bio-based 1,3-propanediol and adipic acid was replaced with the bio-based succinic acid and sebacic acid. Three variations of bio-based polyol were synthesised, and then the most suitable version was validated in a clear coat. Bio-based polyol and coating exhibited properties that were comparable to the properties of synthetic counterparts. Further comparative studies on the properties of synthetic and bio-based polyols are needed. The bio-based polyol has been industrialised, and thus, represents a step forward to the green transition of the coating industry.
The full report, including references, can be found here.